Introduction
High-performance liquid chromatography (HPLC) is a technique used for analysis of liquid mixtures by separating, identifying, and quantifying their constituent components. Liquid chromatography normally operates with smaller amounts of material and seeks to measure the relative proportions of analytes in a mixture. R&D laboratories in the pharmaceutical, food science and oil industries use these instruments for product development or reverse engineering.
Temperature control plays a major role in the liquid chromatography separation process by influencing the interactions taking place between the sample components and adsorbent. Modern HPLC instruments utilize thermoelectric Peltier technology in order to cool or heat the sample tray and separation column. Laird Thermal Systems’ complete thermal management systems optimize liquid chromatography equipment capabilities. Depending on the heat load capacity requirements of the HPLC system, these can be either thermoelectric coolers or thermoelectric assemblies in combination with our SR-54 Series temperature controller.
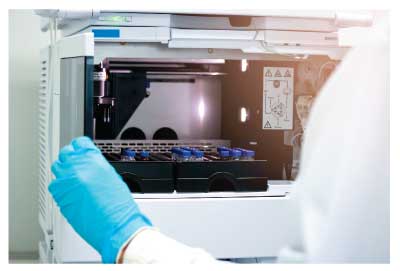
High-performance liquid chromatography use thermoelectric technology to provide temperature control.
Thermal Design Challenges
HPLC manufacturers need to consider several thermal management design challenges including compact form factor vs available capacity, efficiency, cost, and reliability. Additional design factors for use in a Peltier cooling system include ramp rate, temperature stability and robustness to moisture intrusion, which can damage the cooling unit.
Capacity
Even though thermoelectrics are used for both heating and cooling in HPLC equipment, cooling is much more difficult to achieve. When power is reversed to a thermoelectric, the direction of heat transfer reverses, so heat is absorbed from the surrounding environment and added to the resistive heat generated by the thermoelectric cooler. This results in heating efficiencies being several times greater than in cooling mode.
To achieve maximum performance of thermoelectrics, the temperature differential must be accurately calculated to determine how much heat transfer is required. Thermoelectric cooler assemblies generally have a maximum heat pumping capacity rating, which goes down as the temperature differential increases. This rating is similar to air or fluid flow capacity for fan or pumps but the figure can only be achieved under no system impedance.
For a thermoelectric Air-to-Air unit, the hot side temperature is typically defined as the air that heat is being channeled into, which means that the pumping capacity for a standard thermoelectric cooler assembly with a published performance chart is relatively simple to calculate. For an individual thermoelectric, however, the true hot side temperature is not as readily apparent, being dependent on both the amount of heat being moved and the thermal resistance between the thermoelectric hot side and the ambient air. Because of this, the single most critical component of a thermolectric cooler assembly in terms of performane is the hot side heat sink. Because of the added flow from resistive electrical heat generated in the thermoelectric, more heat is transferred across it than across the cold side sink. A 2-3˚C difference in heat sink performance corresponds to a roughly 5% change in the amount of heat pumping capacity.
The actual thermoelectric cooler itself should be the highest rated one (in terms of cooling capacity) that the power budget and heat sink allow. Designers will not be able to select a thermoelectric cooler that uses more current than the system has available, but the heat sink is often the true limiting factor for thermoelectric power. A stronger thermoelectric cooler generates more heat into the heat sink, resulting in a higher hot side heat sink temperature. At lower power, this rise in heat sink temperature may not be enough to make a higher-rated thermoelectric cooler pump less heat than a lower-rated one. As the power gets greater, though, the rise in heat sink temperature puts a larger temperature differential across the thermoelectric cooler, cutting back on its heat pumping capacity. A rule of thumb is, the better the heat sinking design, the further away this transition occurs and the more cooling capacity a designer can squeeze out of the system.
Airflow
Because space is usually a major constraint, paths for incoming and exhaust air must be considered. For cooling of heat sinks, impingement airflow is generally recommended. This is where fans direct air onto the heatsink perpendicular to its baseplate. However, this requires multiple airflow paths, which may not be available in a tight geometry constraint. Another option is to push air along the fin direction, parallel to the heat sink base. However this results in lower performance due to continuous laminar flow with little turbulence at low velocity points. A third possibility is running the airflow normal to the baseplate as in impingement, but have the airmover pulling air away from the base. This is not as desirable as directly flow into the base, as there can be a steady “dead-zone” of low air movement directly under the fan. However, system considerations, such as the need to direct the warmed up heat sink exhaust air away from the tray or electronics, may require such a direction.
For faster response time, the fan can be continuously powered at nominal voltage even between moments when the thermoelectric is unpowered. If the thermoelectric has significant over-capacity and will spend much of its duty cycle unpowered, it may be beneficial to turn off the fan at those moments and extend the system operating life. The air mover is typically the item with the lowest overall lifetime in a thermoelectric cooler assembly.
Sealing
In modern HPLC instruments, thermal management systems are used to provide temperature stability and condensation protection. Moisture penetration into the thermoelectric cavity can lead to premature failure of the thermoelectric cooler and cause system failure. Thermoelectric coolers used in these applications are typically sealed against moisture with either an epoxy or silicone perimeter seal. Good design practice is to have an additional barrier level with insulation gasketing material to protect the thermoelectric cavity in which the thermoelectrics are contained. A gasket of closed cell foam is typically used for this, and yet another barrier level can be achieved by using liquid expanded foam. This makes it extremely difficult for moisture to cross several barriers in order to condensate into the thermoelectric coolers and cause reliability issues.
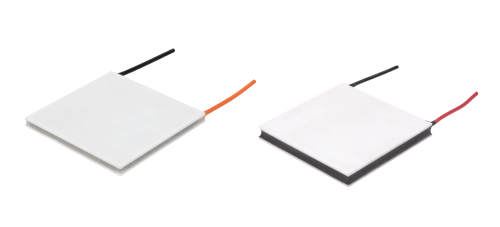
Thermoelectric Coolers used in HPLC equipment are typically sealed with Epoxy (left image) or RTV (right image) in order to prevent moisture intrusion.
Sealing feed passages for power and sensor wiring is also critical. These can be either holes in gasketing or foam or through the solid base heat sink or cold block. In both cases, silicone-type sealant is typically used, though when passing through metal, an appropriate wire sealing grommet is prefered.
Temperature Control
Depending on the temperature control requirements, different design features may be necessary. Precise temperature control ranging from 4 to 40 °C is typically required with heat load requirements ranging from 25 to ~100 Watts. Some new instruments require up to 200 W that accommodate large sample testing throughput. In addition, many new HPLC instruments feature multiple sample storage chambers that have different cooling or heating requirements, increasing the overall system heat load.
Temperature sensing could be installed into the thermoelectric cooler assembly cold plate or nearby. If possible, it is prefered to have the sensing location close to the samples. If the application require temperature stability of more than 1˚C, a temperature controller will be useful. However, if only cooling is needed and the system allows a few degrees hysteresis, thermostatic control will be sufficient. If both heat and cooling are needed, better temperature sensors and some basic PID control logic will be required.
If the user needs to trip an alarm in case the thermoelectric cooler assembly causes a temperature runaway event, a thermostat can be located at the cold block and heat sink base in order to cut off the thermoelectric power. The cause of the runaway event would most likely be due to the failure of a fan used to cool the thermoelectric heat sink.
Thermoelectric cooler assemblies driven by programmable controllers deliver a complete thermal management solution for liquid chromatography systems. A temperature controller provides monitoring and alarm functionality, including i¬dentification of a problematic fan, thermoelectric cooler, over-temperature thermostat and temperature sensor failure — all of which are critical to maximizing instrumentation equipment uptime. Ready-to-use controllers require minimal programming out of the box and can be easily adhered to a thermoelectric cooler assembly or system enclosure. The controller also lowers operational noise, as fans are turned off once the specified temperature has been reached inside the respective HPLC sample tray and separation column.
Thermoelectric Cooling
There are many advantages of using thermoelectrics in HPLC systems, including the ability to both heat or cool with rapid ramp rates. They also provide precise temperature control and are environmentally friendly because they do not use any harmful refrigerants.
The illustration below shows how a typical thermoelectric heater/cooler assembly is used to cool or heat a liquid chromatography instrument. The thermoelectric device is mounted between two air heat exchangers. In cooling mode, air inside the chamber moves across the cold side air heat sink and gets cold. The heat is absorbed by the thermoelectric coolers and transferred to the hot side heat sink. The hot side heat exchanger then dissipates this heat into the air’s ambient environment. In heating mode, the opposite occurs. The hot side fan is typically off in heating mode to limit heat transfer from the ambient environment.
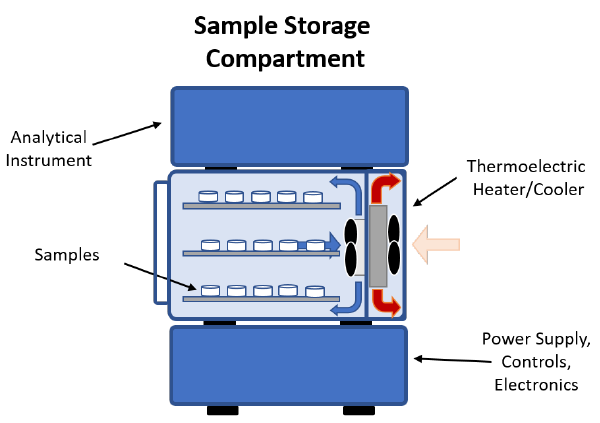
Thermoelectric coolers are active solid-state heat pumps that operate via the Peltier effect. During operation, DC current flows through the thermoelectric cooler creating a temperature differential across the module. While one side of the thermoelectric cooler gets cold (heat absorption), the other side heats up (heat dissipation). Thermoelectric coolers usually connect to forced convection heat sinks to the cold side to absorb heat from inside the cabinet, while the hot side heat sink rejects heat to ambient environment. By reversing the polarity of the device, the thermoelectric cooler can heat inside the cabinet. The ability to both heat and cool allows for precise temperature control in HPLC instruments. Thermoelectric coolers are designed with size, efficiency, cost and continuous reliable operation in mind to enable OEMs to meet thermal design challenges.
Thermoelectric coolers utilize the Peltier Effect to provide precise temperature control for HPLC instruments.
Laird Thermal Systems’ Thermoelectric Solutions
Laird Thermal Systems HiTemp ETX Series is a high-performance thermoelectric cooler delivering superior performance when both heating and cooling is required. This product line uses an enhanced thermoelectric module construction and advanced thermoelectric materials that prevents performance degradation and boosts cooling capacity by up to 10%. By utilizing an improved thermal insulating barrier compared to standard thermoelectric coolers, the HiTemp ETX Series reaches a maximum temperature differential (ΔT) of 83°C.
Offering a wide range of heat pumping capacities and form factors, the HiTemp ETX Series contains over 50 models to support a wide range of applications. Laird Thermal Systems’ robust thermoelectric cooler construction provides superior protection at elevated temperatures where standard grade thermoelectric coolers will fail.
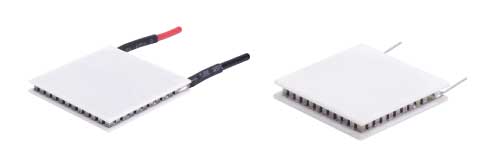
Laird Thermal Systems HiTemp ETX Series
Thermoelectric cooler assemblies utilize thermoelectric coolers in order to either cool or heat depending on sample placement. When DC current flows through the thermoelectric cooler, one side gets hot, while the opposite side gets cold. If you reverse the direction of current flow, then the two sides reverse their function.
Laird Thermal Systems’ Tunnel Series and PowerCool Series thermoelectric Air-to-Air and Direct-to-Air cooling units are designed with efficiency, cost, and continuous reliable operation in mind. Additional design factors include speed of cooling response, temperature stability, resilience against temperature cycling, and resistance to damage by moisture intrusion.
In modern HPLC equipment, Laird Thermal Systems’ thermal management systems are used to provide temperature stability and condensation protection. Depending on the mixture, precise temperatures control ranging from 4 to °C is required. Heat load requirements typically range from 25 to 100W, with some new machines requiring up to 200W to increase sample testing throughput. In addition, many new HPLC instruments feature multiple sample storage chambers that have different cooling/heating requirements, increasing the overall system heat load.
The Tunnel Series thermoelectric cooler assembly offers the most compact form factor with minimal number of airflow paths required to operate efficiently compared to traditional impingement flow thermoelectric cooler assemblies.
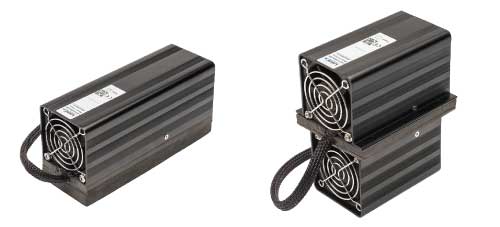
The Tunnel Series thermoelectric cooler assemblies offers a cooling capacity from 10 to 100 Watts.
With a maximum cooling capacity of 280 Watts, the PowerCool Series thermoelectric cooler assembly is designed for HPLC equipment with high heat load requirements. Both the Tunnel and PowerCool Series cool via convection or conduction and utilize custom designed thermoelectric coolers to generate heat-pumping action, which achieve a high coefficient of performance (COP) to minimize power consumption. In addition, the solid-state construction offers low noise operation, long operating life of 40,000 hours MTBF and low maintenance.
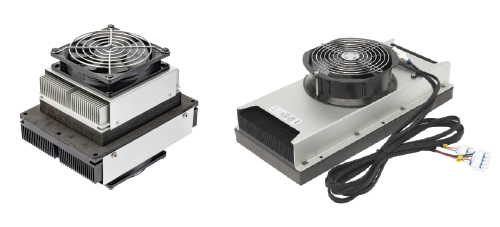
The PowerCool Series Thermoelectric Cooler Assemblies range from 20 to 280 W.
Laird Thermal Systems thermoelectric cooler assemblies are driven by the SR-54 programmable controller to deliver a complete thermal management solution. The SR-54 temperature controller provides monitoring and alarm functionality, including identification of a problematic fan, thermoelectric cooler, over-temperature thermostat and temperature sensor failure — all of which are critical to maximizing instrumentation equipment uptime. The ready-to-use controller requires minimal programming out of the box and can be easily adhered to a thermoelectric cooler assembly or system enclosure. The controller also lowers operational noise, as fans are turned off once the specified temperature has been reached inside the respective HPLC sample tray and separation column.
The advantages of thermoelectric cooler assemblies over other types of thermal cycling devices are precise temperature control, compactness, faster temperature ramp rates and efficiency. With extensive analytical instrumentation temperature control experience, a diverse product portfolio, and a global footprint, Laird Thermal Systems ’ compact thermoelectric cooler assemblies meet the stringent temperature control and mean time before failure demands of analytical instrumentation applications, including liquid chromatography equipment.
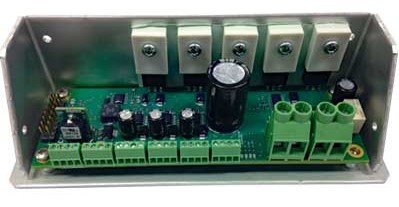
The SR-54 Programmable temperature controller deliver a complete thermal management solution when combined with a thermoelectric cooler assembly.
Conclusion
Temperature control plays a major role in the liquid chromatography separation process by influencing the interactions taking place between the sample components and adsorbent. Liquid chromatography equipment requires thermal management solutions that offer accurate temperature control to optimize the performance. The advantages of thermoelectrics over other conventional technologies are precise temperature control, compactness, faster temperature ramp rates and efficiency. With extensive analytical instrument experience, Laird Thermal Systems provides thermoelectric cooling devices that meet the stringent temperature control and mean time before failure demands of liquid chromatography equipment.
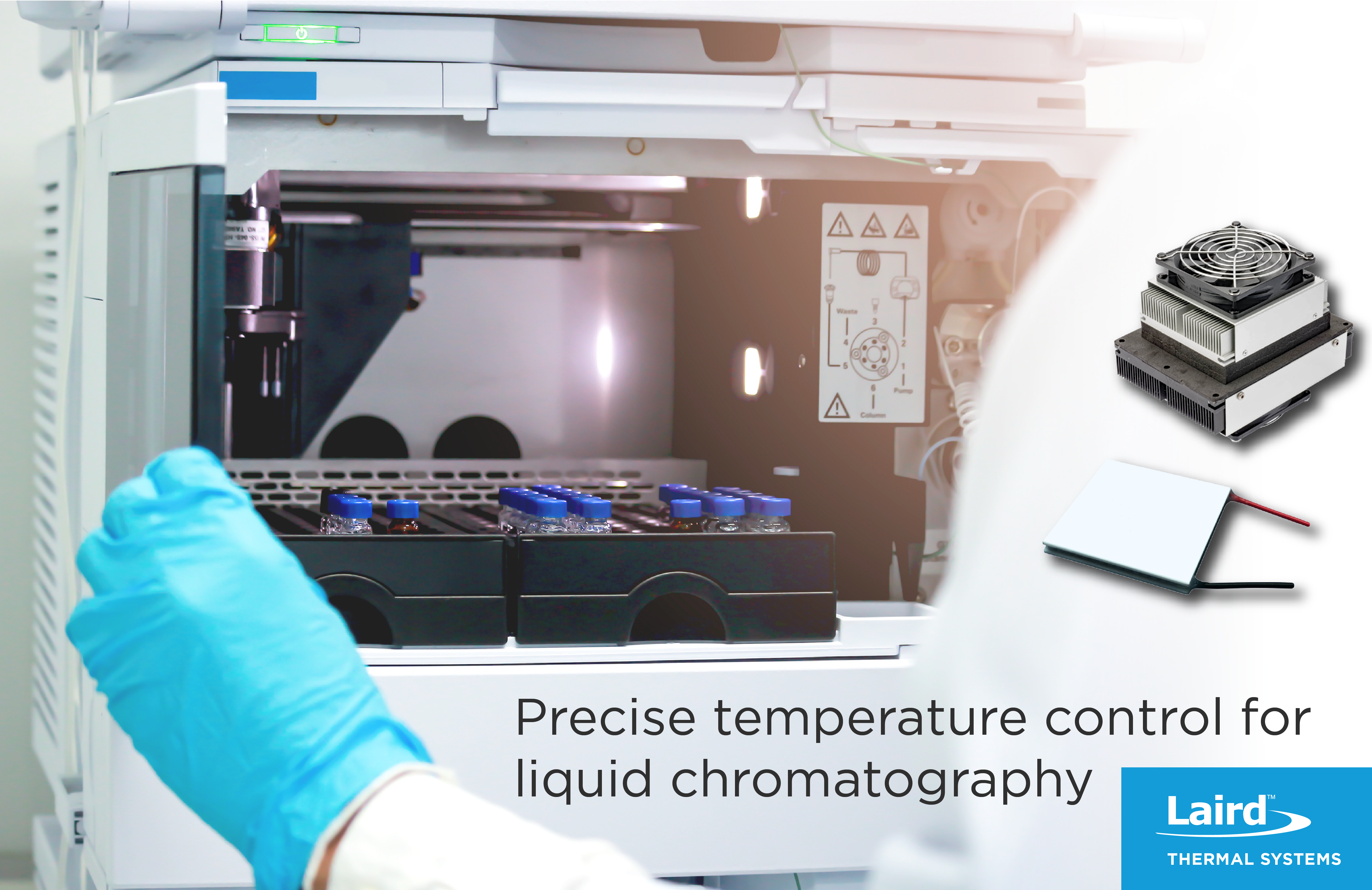